Fluorescence Imaging and Spectroscopy
Our interest:
- Design imaging platform for preclinical and clinical studies
- Design time-resolved instrumentation for preclinical and clinical studies
- Devise quantitative fluorescent analytical model for deeply embedded fluorophores, taking into account photon migration effects, and develop inverse algorithm for 3D reconstruction of fluorophore parameters
Molecular (fluorescence imaging) is becoming an established extension to diffuse optical imaging. This modality offers now much higher specificity than conventional diffuse imaging, it also provides a principally new contrast agent, not limited to the natural absorption changes in tissue, resulting from blood volume/oxygenation variations, that are the typical aim of NIRS imaging, but much broader set of molecular targets, e.g., changes in metabolic/functional activities of the cells, including expression of specific genes, etc. It should be noted that changes in the scattering and absorption properties can be minor or not specific, especially at the early stages of the disease or during the treatment monitoring. In such cases fluorescence imaging, using highly specific molecular probes, can provide the missing information, helping to detect/monitor the disease progression. Additional diagnostic information can be obtained from analysis of the fluorescence lifetime measurements, because for some fluorophores the lifetime is sensitive to their environment (e.g., pH, temperature etc). Fluorophores with known lifetime dependence on pH and/or temperature can be used for functional imaging and diagnosis of malignancies.
A time-resolved small animal molecular imaging system was developed that uses near-infrared contrast agents to visualize in vivo distributions of fluorescence intensity and lifetime inside a turbid medium. The system was used to collect data by scanning a measurement head which consists of a linear fiber array with fixed separations between a single source fiber and several detection fibers over a two dimensional region of interest (ROI). Using several separations between the source and detectors allowed us to probe different depths of the medium more efficiently. We obtained experimental results from tissue-like phantoms as well as in vivo studies of mice tumors to validate the system for future applications of minimally invasive functional imaging.
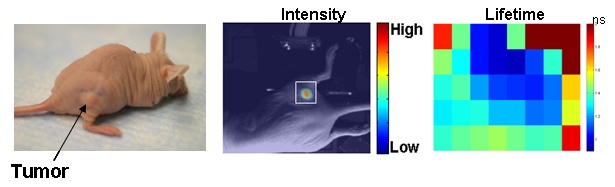
Due to highly scattering properties of tissue in the visible and near infrared wavelengths, adequate inverse algorithms to reconstruct spatial and temporal characteristics of the deeply embedded fluorophores are required. Transition from preclinical studies, dealing with superficial tumors (xenografts) in the mouse model, to clinical studies on breast tumors deep inside turbid tissues would require a more sophisticated analysis of the photon migration. The general inverse problem of diffuse optical tomography, including fluorescence tomography, is ill-posed, making it computationally extensive while reducing the accuracy of quantifying the optical parameters. Our specific aim is to explore possible approaches to fluorophore localization inside the turbid medium and quantification of the fluorescence lifetime, excluding effects of photon migration. We have also started development of more general inverse algorithm, based on Diffuse Optical Tomography (DOT), to reconstruct 3D distributions of optical parameters, using numerical models of photon migration, based on "local" characteristics of the Temporal Point Spread.